About Our Lab
About the TNT lab and active projects
About Our Lab
The Traumatic Nerve Technologies (TNT) Lab conducts research in many diverse areas. We're taking a multidisciplinary approach to understanding traumatic injuries, cell repair strategies, and technologies that assist in prevention, identification, and treatment of injured tissues.
By advancing the fundamental understanding of the behavioral and molecular consequences following traumatic injuries, we will further identify targets and outcome measures needed for effective treatment strategies.
Traumatic brain injury (TBI) is a large focus of our work and represents a substantial clinical burden worldwide. Patients that sustain a TBI are at greater risk of developing neurodegenerative diseases, in addition to experiencing cognitive and psychological deficits following injury. Few therapeutic strategies have proven successful in mitigating the long-term effects of TBI. This issue is largely attributable to the heterogeneity of mechanical insults that cause TBI, a lack of mechanistic understanding of the cell response to trauma, and failed translation of therapeutics from pre-clinical models to clinical trials.
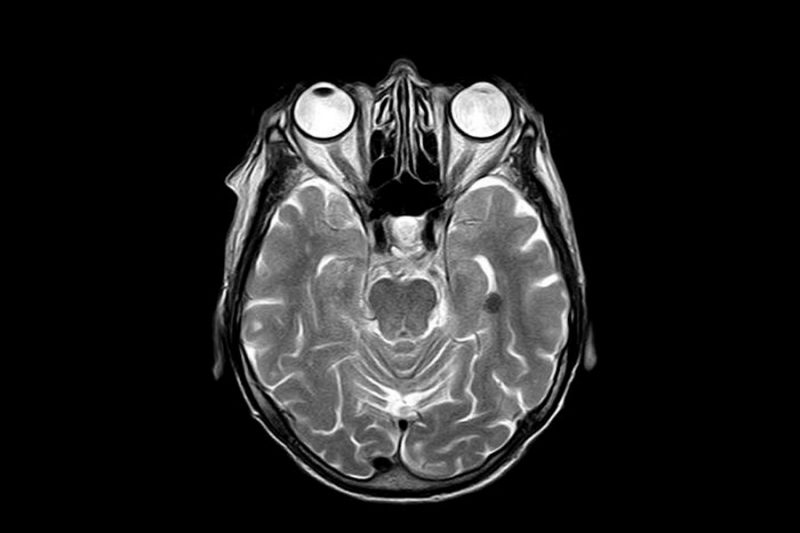
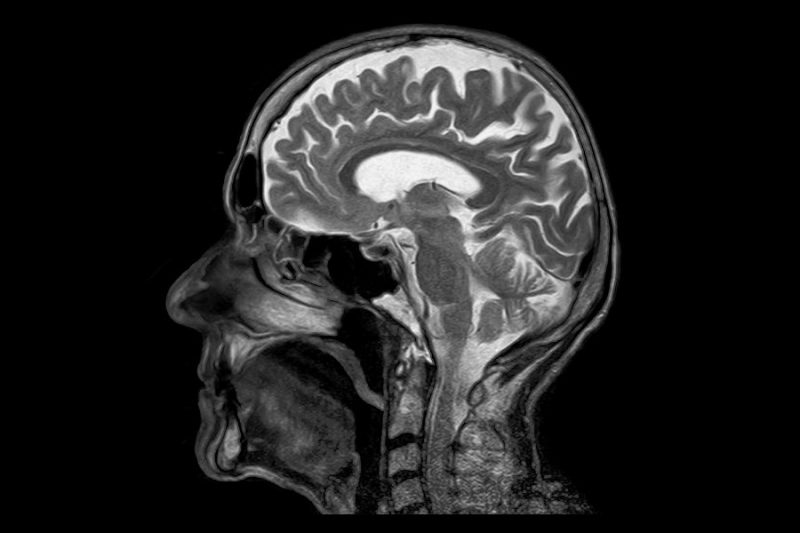
About Our Research
An area of focus is on Veterans. A significant number of Veterans returning from military conflicts are suffering from closed head injuries due to blast exposure. Blast exposure is a common cause of injuries in military conflicts and often leads to blast traumatic brain injuries (bTBI). To further complicate the injury, combat personnel can be exposed to multiple low-level blasts, which could lead to long-term sequelae.
Increasing numbers of Veterans are returning from deployment suffering from bTBI. A large number of these Veterans are exposed to repeated concussive injuries which may lead to long-term neurological impairments. Since bTBI has become a leading cause of disability worldwide, there is a critical need for a greater understanding of the injury mechanisms and long-term neurological dysfunction following single and multiple blast exposure(s)/concussive injuries. Models allow for in-depth examination and evaluation of such exposures and injuries and provide groundwork for later clinical studies involving human participants, further highlighting the importance of and need for this proposed research.
Current Neurotrauma Projects
A critical part of mitigating potential injury, attending to sustained injuries, and treating sequelae is understanding how mechanical factors damage the body. It is known that exposure to blast waves can injure tissues. Due to the variability in blast exposures, it is critical that we understand the biomechanical and material response of tissues using an integrated biomechanical approach, from macroscopic to microscopic levels.
Our multidisciplinary team of engineers and scientists explore this transformation of energy into injury. Using state-of-the art facilities, our team investigates the challenging questions of the insult turns to injury. Projects use established in vitro, preclinical, and surrogate models for clinically relevant applications. Our blast simulators allow us to investigate the interactions between blast waves and biological tissue.
We collaborate with sponsors to develop a wide array of technologies, including computational models, clinical therapies, personal protective equipment, sensors, and biofidelic surrogates, that advance the field’s ability to protect from blast-related injuries.
To decipher cell injury and repair mechanisms, neurotrauma studies have traditionally focused on neurons. However, mechanical insults, such as traumatic brain injury (TBI), lead to perturbations in cellular communication and neural networks involving glial cells and their precursors. Thus, research interests have shifted to the significance of glial cells (astrocytes, microglia, oligodendrocytes) and their role in the progression of and recovery from TBI. Our approach is a combination of computational and experimental tools that provide unique but complementary data to help better understand the fundamental biological mechanisms.
Current works are exploring the distinct role astrocytes play in TBI progression, oligodendrocyte precursor cells (OPCs) response to mechanical insults, and microglia activation and recruitment. Studying glia’s fundamental capacity and response to injury are important targets in the way of developing effective treatments. The projects incorporate aspects of cell biology and mechanics with signaling networks and computational biology modelling to understand how force transduction via cell-matrix interactions may contribute to complex sequelae that occur after high-rate insult associated with injury across multiple species. This work will create a platform by which we can analyze relevant molecular relationships and potential therapeutic targets in relevant preclinical brain injury models.
Complex traumatic brain injury (TBI) induces distinct pathologic influences on brain regions that lead to acute and persistent behavioral symptoms. The neurobehavioral sequelae of TBI may be partly linked to neuroplastic changes that occur in response to mechanical injury and associated stress. Our goal is to expand basic mechanistic insight into TBI-induced epigenetic and molecular signaling effects on regions such as the amygdala and connecting neural circuitry, with a long-term goal of identifying new molecular targets for future drug development.
This multidisciplinary approach combines epigenetic, histologic, and behavioral assessment techniques to broaden and better understand the mechanistic underpinnings that govern neuroplasticity and aberrant neural circuitry of TBI-induced behavior. Linking broad neuromolecular alterations to distinct neurobehavioral phenotypes will help transcend interspecies differences and improve the translatability of acute neurotrauma findings to the clinical setting. Most importantly, this work will establish new pathologic molecular profiles that will guide future mechanistic investigations and identify novel molecular targets that will facilitate potential diagnostic and pharmacotherapeutic development.
The TNT Lab also hosts projects that explore the multiple clinical symptoms of TBI.
- Headaches: One common aliment is chronic pain, most commonly chronic post-traumatic headaches. Suffering from chronic pain conditions can impact a person’s overall health and quality of life as it can lead to psychological issues, cognitive deficits, and cause setbacks in functional recovery. Therefore, there is a need to study the link between TBI and the development of chronic pain behavior in preclinical models.
- Anxiety: Behavioral studies have linked anxiety development with TBI. Stress, which refers to a combination of physiological, neuroendocrine, behavioral, and emotional reactions to a stimulus, is a defensive adaptation under physiological conditions. We study the combination of stress and TBI to better understand the influence that these conditions have on the development of anxiety.
- Depression: Depression is among the most frequent complaints of those with persistent symptoms stemming from TBI. Glutamate has been identified as a possible link between TBI and depression. Our lab is examining the chronic downregulation of glutamate transporters and receptors in the brain following TBI to uncover a possible molecular target for treatment.
- Risk-taking behaviors: Disinhibition is a behavioral trait common to those following those with repeated concussions and is associated with risk taking, impulsivity and aggression frequently leading to maladaptive behaviors (e.g. substance abuse). Our lab is studying the influence that TBI has on the development of these risk-taking behaviors to help identify effective strategies to mitigate their development.
Traumatic brain injury continues to be a serious problem in society with over two million occurrences annually in the United States. Currently there have been no neuroprotective drug trials to survive past Phase III clinical trials. Previous in vivo testing involves injury models that are not clinically relevant, do not include biological variability, and are typically small animal models. There is a need to study TBI in gyrencephalic models using novel injury devices that simulate human injury conditions.
Complex MR and PET imaging techniques used in parallel with cognitive/behavioral tests can help measure changes compared to pre-injury assessments and longitudinally to evaluate possible recovery caused by TBI. Histology defines the underlying neuropathology. This data can ultimately be used to develop injury thresholds for human conditions. Cognitive impairments and novel imaging modalities can relate underlying damage to make a link between noninvasive and invasive measurements. These measures can also help ease translation to clinical tools to improve diagnosis and intervention.
Further, addressing biological variability and sex differences will help address some of the limitations in current preclinical models. Ultimately, developing a standardized preclinical model that produces clinically relevant TBI in a gyrencephalic model will better measure drug safety and efficacy. In addition, a well-characterized model can give insight into new drug targets that were missed by previously used in vivo models with non-realistic injury conditions.
No neuroprotective or restorative drug trials have extended past Phase III clinical trials but the demand for TBI treatment only increases. Thus, novel treatment strategies are needed to improve the outcome of those affected by TBI. Cranial osteopathic manipulative medicine (cOMM) may be an alternative to traditional treatment approaches and involves the gentle application of manual force to the head and the axial spine. This therapy releases soft tissue restrictions and enhances fluid flow. Using preclinical TBI models, we examine the efficacy of cOMM to influence molecular, physiological, and behavioral change. Our long-term objective is to determine the physiological response of the injured brain to cranial OMM as a treatment strategy.
The TNT Lab also hosts projects that explore the application of cOMM on Alzheimer's disease, which is the most common form of dementia and affects about 6.7 million individuals in the United States. Individuals with Alzheimer’s disease experience an increase in harmful proteins such as amyloid Beta. Like TBI, there is a lack of physiological and pharmacological methods to increase fluid circulation, but cOMM may prove to be an effective strategy. By implementing PET imaging, animal behavior assays, and molecular analyses, we aim to further our understanding of cOMM and the benefits that may come from the treatment.
Department
The Virginia Tech Department of Biomedical Engineering is a unique multidisciplinary interface between engineering and medicine applied to real-world challenges to enhance the quality of life. Our world-class faculty and students innovate and discover across a continuum of systems, from natural to engineered to medical.
Affiliations
The TNT lab is affiliated with the Center for Injury Biomechanics, the Institute for Critical Technology and Applied Science, the Virginia Tech Transportation Institute, and the Virginia Tech - Wake Forest University School of Biomedical Engineering and Sciences.
Funding
Funding for lab research comes from the U.S. Department of Defense, U.S. Department of Veterans Affairs, National Institutes of Health, and the National Science Foundation.